Hanford
The little town of Hanford, from which the site takes its name, was
founded well over 100 years ago, as one of several small ranching communities in
the area. It ceased to exist in 1943, when the few residents were ordered to
move, by the U.S. government. The town of White Bluffs was also vacated. The
resulting Hanford site
encompasses approximately 600 square miles, and is adjacent to the Hanford
Reach, a protected preserve. This is similar, in many respects, to what happened
a couple of thousand miles away, in Tennessee, where residents of the town of
Wheat, and of several other small farming towns, were ordered to move, to clear the
way for the building of the various plants which would make up the Oak Ridge
site, as well as the city of Oak Ridge itself. In both cases, these similar
actions were taken for similar reasons.
When America was
struggling to develop what was then called the atom bomb, before the Germans or
Japanese could do so, locations were required which had a rather unique
combination of features. All had to be large, open, have access to large amounts
of electricity, be sparsely populated, and be isolated enough to be kept secret,
and to shield the public from any possible dangers.
Few such places existed then, or now. Generally, ample electricity is
associated with population centers. Oak Ridge was begun first and was initially
planned as the site of the entire operation. Soon enough, though, it became
plain that the development of the atom bomb was to be a much larger project than
had originally been envisioned.
The use of uranium was initially
thought to be key, as a nuclear explosive; but it was recognized that only a
massive industrial effort would be able to separate the required isotope, from
the large amounts of natural uranium being refined. The largest building in the
world was built at Oak Ridge, to do the separating. The K-25 separation building,
at Oak Ridge,
was a half mile long, by a quarter mile wide, and took over 2 years for 20,000
construction workers to complete. On top of all of this, it took huge amounts of
power, and considerable time to separate the required isotope. There had to be a
better way. Despite all of the time, effort, and money invested, K-25 did not
produce any appreciable amounts of nuclear explosive in time for WWII - a better
way had been found.
The better way turned out to be
a sort of a nuclear shortcut, in the form of the then mysterious element 94.
Element 94 does not exist in nature, at least not here on Earth (actually,
recent discoveries have turned up minute amounts of certain isotopes; but only
in microscopic quantities), and had never
been seen by man, before small quantities were produced as a byproduct of
certain nuclear reactions. These small quantities of this new element showing
up, opened new potentials, and a possible different approach. Scientists
calculated that a common isotope of this new element would make a great nuclear
explosive, better in some ways than the difficult to separate uranium 235
isotope. It also seemed that, once proper facilities were put in place, it could
be produced and purified more quickly than the uranium isotope, because it could
be separated by relatively simple chemical means, rather than the painstaking
and energy intensive weight separation employed in obtaining uranium 235. The
new substance would be named plutonium.
The air cooled X-10 reactor was
built at Oak Ridge, specifically to see if this new substance could be produced
more quickly, and in quantity. The idea proved both viable and practical; but a
larger reactor was required. The X-10 (in diagram to the right) was a 24 foot block of graphite, which
produced around 1000kw, using 1248 loading tubes. The rule of thumb about
plutonium production is 1 gram per MW, per day, so the X-10 produced a gram of
plutonium a day. This was a good demonstration of the plausibility of the
concept, and would be the blueprint followed in future production reactors; but
it was not nearly enough. At such a rate of production, it would take 16 - 18
years, to make enough for a bomb. Something bigger was needed, by a whole
magnitude of order, and that was the problem.
The Manhattan Project was
getting quite a bit bigger than anyone had expected, which was a particular
problem with the level of secrecy being sought. The war time population of Oak
Ridge was about 70,000 people. There was the X-10 facility, the calutrons at
Y-12, and the huge K-25 separation plant, as well as the city itself, associated
labs, and support facilities. The new
plutonium based approach would require an effort equal to that already in place
for creating a uranium based bomb. Oak Ridge was getting too crowded to base
such an effort here. A new site would have to be found.
One of the locations scouted
turned out to be ideal. There were few residents, it was miles from any major
population center, it was desolate and almost unpopulated. The nearby dams
produced abundant electrical power, and as a bonus the rivers which fed the dams
offered a generous supply of cooling water for the proposed reactors. It was
perfect. Located in south eastern Washington state, it was far enough from the
coast, that there was little worry about Japanese attack. The residents were
moved out, being given thrity days notice, and the military and scientific teams were moved in.
The new reactor, known as the B
Reactor, was to be water cooled, rather than relying on the less capable air
cooling of X-10. This, along with its increased size, would permit much more
power, and thus much more plutonium production. The initial power level attained
was 250 times that of the old X-10 reactor. At this level of production, it
would take months, rather than years, to produce enough plutonium to complete a
bomb. A year would be enough time to produce plutonium for use in ten bombs,
though in reality the Manhattan project only produced four bombs, two of which
were dropped on Japan, and one of which was set of in the Trinity test.
The reactor was not built here
in isolation. Two other reactors, the D and F, were built before the war ended.
With B, F, and D reactors built during the war, and C to be built latter,
the question has been
raised about the A reactor, and apparently there was none.
(Perhaps X-10 was considered to be the A
reactor. Who knows? I have never heard anyone think to ask what ever happened to
reactors X-1 through X-9.) There was also far more to the site than the eventual
nine reactors.
Still, the reactors were only the beginning of the process and of the
project. Plants were needed to produce the nuclear fuel, and purify the end
product. It was also planned that there would be more than one reactor here, as
the site
was such an ideal location. There would need to be waste disposal sites,
and laboratories. The further along the project went, the more obvious it became
that this would not have fit at Oak Ridge, with the facilities that were already
there. During the war, 48,000 people lived and worked at what had originally
been a desolate stretch of ranchland and desert. The Hanford site has a list full of
interesting facts, that show just how impossible it would have been to crowd
these facilities in with those already at oak Ridge. As an example of the
logistics, they say that 6500 eggs were used in a typical Sunday breakfast, along with
5000 pounds of sausage - 120 tons of potatoes were eaten each day, along with
30,000 doughnuts, and 8000 pounds of coffee.
The one and only reason for all
of this effort was the production of plutonium. After WWII was won, the United
States pursued development of nuclear weapons, as a necessary part of having
become the new world power. When the Cold War started soon afterwards, with
America and the Soviet Union becoming the super powers, there was even more
reason to step up plutonium production, in order for America to continue to be
the new world power. Eventually, nine reactors would be
working here, to produce over two thirds of the nation's stock of plutonium. B
reactor was shut down in February of 1968, with the similar single pass reactors
all shut down by 1971. The N reactor, which was a multi pass reactor, remained
open until 1987. The N reactor was the only Hanford reactor used to produce
electricity.
A single pass reactor uses
cooling water only once. In the case of Hanford, the water was taken from the
river, cycled through the reactor, and then discharged into a settling pond,
before being emptied back into the river. A multi pass reactor uses the same
water over and over again, in a closed system. Unfortunately, single pass
reactors can pollute a river, particularly when water is not held for sufficient
amounts of time in the settling ponds, which can often occur when there is
demand for increased production.. The multi pass reactor has no such
issues.
The main components of the site,
besides the nine reactors, were the chemical separation plants, called
canyons. The first was nicknamed Queen Mary, because of its huge size, and the
way it appeared on the desert horizon, like a ship on the ocean - complete with
stacks. These were located in the 200 areas shown on the map above, while the
reactors were along the river in the 100 areas, about 10 miles away. These were
connected by a rail line. There were also five reprocessing plants, and a
finishing plant, which was able to convert the results of the separation plants
into pure plutonium metal. There were also plants for fuel production.
Hanford Today
Today, much of the old Hanford is gone, and
production of plutonium here has ended. This does not mean that the site is
inactive - quite the contrary. A huge, two billion dollar a year clean up is
going on, and will continue for the next thirty years or so. If the economy is
bad in your area, and you are looking for a new start, there is quite a bit of
opportunity here. If I were a younger man, I might just consider migrating here
myself. Men and women will
make entire lifetime careers doing this important work, One major aspect of this
clean up is the sealing (called cocooning) of the old production reactors. These
are in the 200 square mile river corridor area, also known as the 100 area. A
cocooned plant is partially dismantled (up to the shield wall), welded shut,
sealed with concrete, and capped. Every ten years or so, the welds are broken,
and survey teams go in, to measure residual contamination. The photo to the left
shows the cocooned C reactor, next to the B reactor that I visited. This was to
be the fate of the B Reactor, and would have been, were it not for the efforts
of various preservation groups.
There were other facilities here, including fuel fabrication plants, which
will need clean up, and have largely been demolished; but the reactors are the main concern.
According to DOE, the river corridor contains 761 sites, where clean up is
required. This includes the reactors, the fabrication plants, landfills, waste
storage tanks, cooling ponds, and the residue from leaks and spills. During The
Cold War, such things were a concern, but a secondary concern, when compared to
winning a possible nuclear war with the Soviets. Today they are the
primary concern. Ironically, places like Hanford saved us from the nuclear
threat posed by the Soviets, and other enemies. Today, clean up efforts seek to
save us from the nuclear threat posed by these very saviors.
Most of this waste, particularly contaminated soil and low level
waste, will be relocated to the Environmental Restoration Disposal facility
(ERDF), within the Hanford Site
itself. This is on the central plateau of Hanford. ERDF
has a capacity of 10 million tons of material. Once filled, it will be capped,
and barriers will be put in place to prevent leakage. The radioactive
contamination will then be allowed to decay, safely contained. The ERDF was one
of the stops on the site tour, and the place is absolutely huge. Our guide
boasted that the great trench can be seen from space, with the naked eye. As we
watched, a stream of trucks shuttled materials down into the trench, to be moved
into position by bulldozers, and other heavy machinery. And so it will go, for
decades before completion.
The river area could be cleaned up within the next 5 - 10 years, and
possibly be opened to the public and integrated within the Hanford Reach
preserve.
Still, not everything has been cleaned up, and not all sensitive research
has been stopped. Many of the areas of the site are still under heavy security,
and will continue to be so during the foreseeable future.
Also stored at the site, are
2100 tons of spent fuel which was awaiting processing when production was shut
down, as well as another two tons of spent fuel from commercial reactors, and
another 140 tons of uranium which was to become fuel. Nine hundred tons of
uranium have been removed from the site, presumably to Yucca Mountain or some
such place. One of the most interesting things that I saw (but sadly was unable
to photograph), was a trench containing discarded reactors from decommissioned
nuclear submarines and surface ships. There were dozens of them in a long
trench - perhaps a hundred or more. Most are brought here from the Bremerton shipyards at Seattle. They
appeared as sections of huge metal cylinders.
At the other end of the site, in
the 300 area (see map above), most of the older buildings have been demolished.
This was largely where fuel was fabricated, and most of the buildings were too
contaminated to be saved.
There are still
radiological labs here, and much research is being done. Some new facilities
have been constructed, and the Pacific Northwest National laboratory is still
actively doing research.
Nearby is the Ariva plant, where commercial nuclear
fuel is still being made. This is a pretty secure place; but it is good to know
that there is still something of a commercial nuclear infrastructure in this
country.
Energy Northwest does have one power reactor here. This is the only active
reactor currently at the site. Another reactor, a smaller unit known as the Fast
Flux Test Facility, was also in place; but sadly was decommissioned and is being
dismantled. This was a sodium cooled reactor, which used fast neutrons, and was
a test bed for new technologies.
Plutonium
Production
At its original output, the B Reactor could produce 250 grams of
plutonium a day – about ten ounces. Eventually, the B reactor would produce
eight times this amount, with some other reactors making over twice as much. This
sounds far simpler and more straightforward than it really was, as if you might
just find bits of plutonium laying at the bottom of the rector; but there were a
couple of complications. At its peak, in the sixties,
Hanford
probably produced enough plutonium for 30 – 35 nuclear warheads, every week.
The only way to get that plutonium was to shut the reactor down, and push
the fuel out into the collection area. The fuel would then be allowed to cool,
after which it would be collected for processing. So, how often do you do this?
Well, that was the big question.
Recall that the B Reactor holds 200 tons of fuel, out of which around ten
ounces a day is eventually transmuted into plutonium. 200 tons comes out to
6400000 ounces. If you do the math, it turns out that it would take 1753 years,
for the whole mass to be converted. Even at it’s maximum output of over 2GW, it
would still take close to 500 years to convert the whole mass. We didn’t have
that much time – there was a war on. Even if we did have the time, there is
another factor.
Just for argument’s sake, lets say that you did have 500 years to wait
around for all of your uranium to transmute into plutonium. So you leave those
rods in the reactor for 500 years, and then have your descendants push them out.
Would you have 200 tons of fully converted plutonium 239? Certainly not! What
you would have, would be a mess - an incredibly dangerous, and useless, mixture of highly
radioactive transuranic elements, and radioactive isotopes. Even the proportion
of plutonium included in the mixture would no longer be pure Plutonium 239; but
would be of several different isotopes, and would be useless without the same
sort of separation which presently makes U235 such a difficult material to
procure. The reason for this is simple.
Inside of a nuclear reactor, elements are being constantly transmuted.
This includes the element of plutonium. Plutonium is not produced directly. What
happens is that the uranium 238 is converted to Uranium 239, which quickly
decays to Neptunium 239, and then to Plutonium 239 – the desired end product.
Unfortunately, all of these elements are subject to further transmutation, as
long as they remain in the reactor.
So at any given time, you would have a mixture of uranium 238 – the
original fuel – along with some uranium 239, neptunium 239, and plutonium 239.
You would also have various isotopes of iodine, krypton, xenon, and americunium,
as well as unwanted isotopes of plutonium. These elements have different half
lives, which is to say, they transmute at different rates. They also have
different capture cross sections, which is to say they are not all affected by
the neutron bombardment, which occurs inside of a reactor, at the same rate. The
proportion of these elements thus changes over time. This variety of elements is
the flip side of the nuclear shortcut which allows plutonium to be produced to
supplant uranium as a nuclear explosive.
Though we humans see uranium 238 as fuel, and plutonium 239 as the end
product, nature has no such bias. Plutonium 239 which remains in a reactor,
continues to fission or capture under neutron bombardment, and can contribute as much as a
third of the energy to the output of a reactor. In a power reactor, this is
great news, and led to the idea of the breeder reactor. In a production reactor, it is a terrible nuisance. You don’t go
through all of the trouble to produce this plutonium, just to have it burn up
inside of the reactor, or become contaminated with unwanted isotopes. So you have to get it out; but at what point?
Well, I can’t really answer that question. It appears to be a matter of a
few weeks at most; but I am unable to find a definite answer anywhere on the
web, or in any of the books on the subject. I may have just not researched
thoroughly enough, or the information may simply not be available. The subject
may still be classified, as it would seem to be a useful bit of information to
potential nuclear states. There is, presumably, a point at which the amounts of
useful materials (uranium 239, neptunium 239, and plutonium 239) being produced
approaches the law of diminishing returns, where as much (or more) is being
burned up as is being produced. It may be that, even before this point is
reached, you may wish to eject the fuel, in order to save your fuel stock. There
is also a concern about producing undesirable isotopes of plutonium, which could
not be easily removed.
According to
the World Nuclear Association (
http://www.world-nuclear.org/info/inf15.html )
"It takes
about 10 kilograms of nearly pure Pu-239 to make a bomb. Producing this requires
30 megawatt-years of reactor operation, with frequent fuel changes and
reprocessing of the 'hot' fuel. Hence 'weapons-grade' plutonium is made in
special production reactors by burning natural uranium fuel to the extent of
only about 100 MWd/t (effectively three months), instead of the 45,000 MWd/t
typical of LWR power reactors. Allowing the fuel to stay longer in the reactor
increases the concentration of the higher isotopes of plutonium, in particular
the Pu-240 isotope. For weapons use, Pu-240 is considered a serious contaminant,
due to higher neutron emission and higher heat production. It is not feasible to
separate Pu-240 from Pu-239. An explosive device could be made from plutonium
extracted from low burn-up reactor fuel (i.e.
if the fuel had only been used for a short time), but any significant
proportions of Pu-240 in it would make it hazardous to the bomb makers, as well
as probably unreliable and unpredictable. Typical 'reactor-grade' plutonium
recovered from reprocessing used power reactor fuel has about one third
non-fissile isotopes (mainly Pu-240)."
One of our guides had worked as a reactor operator, and he advised us
that refueling the reactor occurred at an intervals of a few days, to a few
weeks, which differs a bit from what the World Nuclear Association says. He also advised that it was not necessarily done en mass. The fuel in the
center portions of the reactor, where the radiation was more intense, and the
reactions more vigorous, was cycled more often than that at the edges. I tend to
place more credence in the words of the reactor operator, mainly due to his
first hand experience. The World Nuclear association is more about nuclear
power, than nuclear weapons. Using
this as a guide, just to get an idea of what is involved; let’s create the
following vastly simplified (and possibly numerically incorrect) scenario:
After two weeks (14 days) the reactor is stopped, and the fuel ejected
for processing. It sits in a water filled receptacle at the back of the reactor
for a few days. This permits it to cool, and also allows the existing Uranium
239, and Neptunium 239 to transmute into Plutonium. Let’s assume the B reactor's
maximum power of 2GW, which was about eight times its initial operating power. So it would
have produced, assuming no losses to further transmutation, 1120 ounces of
plutonium, or about 70 pounds – out of 200 tons of material. The actual amount
would be less due to further transmutation by continued nuclear reactions. The
longer the material is left in the reactor, the greater these losses would be.
A crane operator removes the rods, and places them in buckets for transit
to the processing plants, about ten miles away, by a rail line built for this
purpose. So once you have the rods out of the reactor, and have dissolved them,
a series of chemical steps separates the plutonium, which differs chemically
from the original uranium feedstock. What you end up with is about 70 pounds of
plutonium (enough for maybe five nuclear warheads), and around 200 tons of left
over uranium dissolved in hundreds, or thousands of gallons of solvents and
other noxious chemicals. These tended to be stored in gigantic tanks; but even
this was not the end.
Uranium is a strategic material, and even though un-enriched uranium is
not particularly valuable, it is not something you simply want to throw away. It
is also not something that you want to leave just laying around. So, various methods
of reclaiming the dissolved uranium were developed. Some worked better than
others. All required some pretty serious, and dangerous, chemistry. Reclaiming
the uranium, still left the chemicals from which it had been separated, as well
as the additional chemical residue from the process of reclamation. More storage
tanks were built. And on it went, though much of The Cold War.
So this is the legacy that we are now cleaning up. There is everything
from hexane and chromium, through leaky tanks of plutonium slurry, to barrels of
oil with shavings and scraps of uranium. Thrown in the mix are partially
processed spent fuel rods, and partially finished new fuel rods. Often, when
excavating an old land fill, the workers have no idea what they will find buried,
making this a very slow, careful, and painstaking process.
Reactors at Hanford
Reactor
|
Start-up
|
Shutdown
|
Initial power
(MW)
|
Final power
(MW)
|
305 Test Pile |
Mar 1944 |
|
|
|
B-Reactor
|
Sep 1944
|
Feb 1968
|
250
|
2210
|
D-Reactor
|
Dec 1944
|
Jun 1967
|
250
|
2165
|
F-Reactor
|
Feb 1945
|
Jun 1965
|
250
|
2040
|
H-Reactor
|
Oct 1949
|
Apr 1965
|
400
|
2140
|
DR-Reactor
|
Oct 1950
|
Dec 1964
|
250
|
2015
|
C-Reactor
|
Nov 1952
|
Apr 1969
|
650
|
2500
|
KW-Reactor
|
Jan 1955
|
Feb 1970
|
1800
|
4400
|
KE-Reactor
|
Apr 1955
|
Jan 1971
|
1800
|
4400
|
N-Reactor
|
Dec 1963
|
Jan 1987
|
4000
|
4000
|
FFTF |
1982 |
1992 |
400 |
400 |
Some photos of the Site
I am only guessing at what some of these are. if you happen to have worked
at Hanford, or just happen to know what some of these places are, I would
appreciate hearing from you. I felt a bit like an old time Soviet spy, grabbing
these photos from the bus window, as we skirted the site. No doubt, large
numbers of photos were taken by actual Soviet spies during the Cold War.
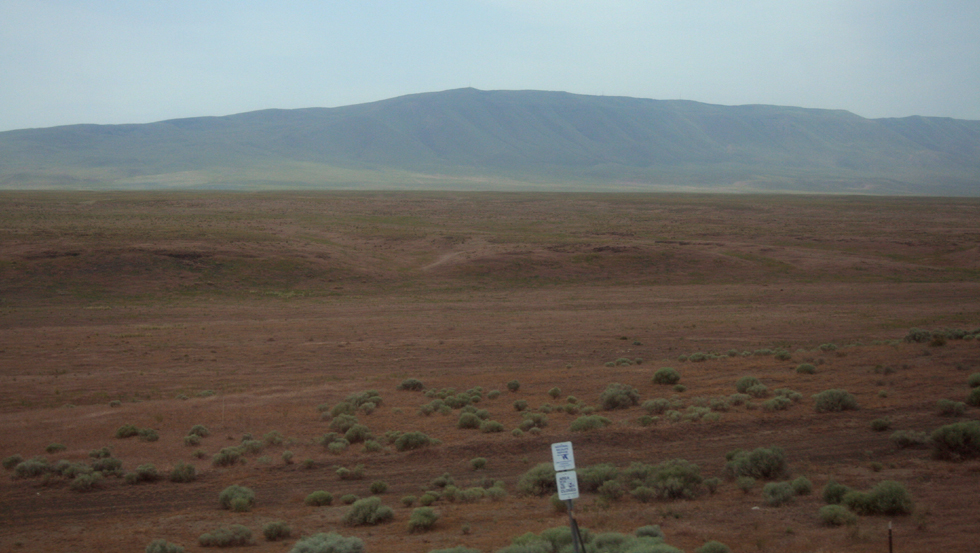 |
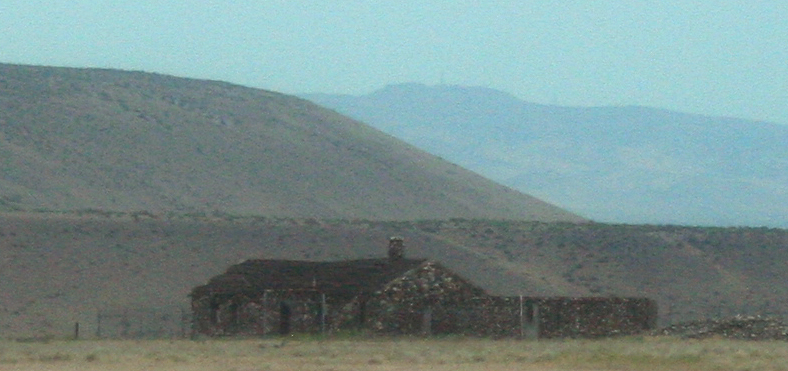 |
Above:
Hanford as it probably looked for thousands of years, before being taken
over the the government.
Left:
An old homestead, probably a ranch, from the area's early days of
settlement.
Below:
A view of the Columbia River, from the road outside the site. Reactors
are visible along the river. |
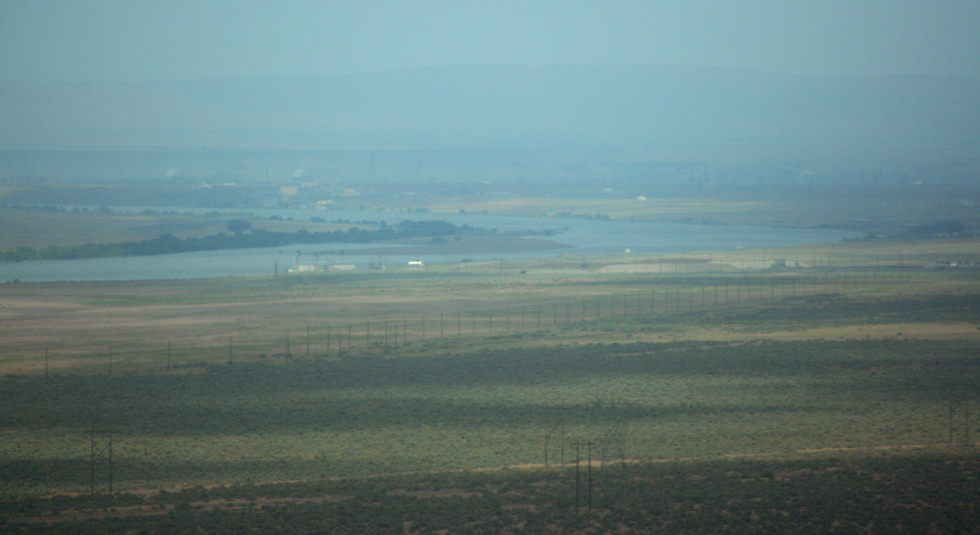 |
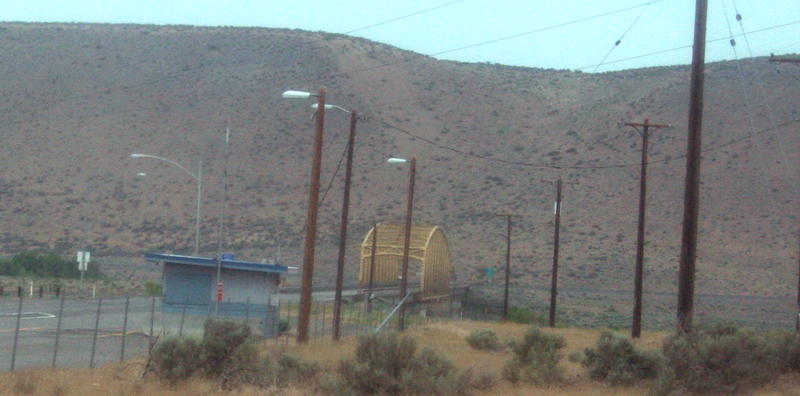 |
An old guard shack, as well as an iron bridge, harken
back to WWII. |
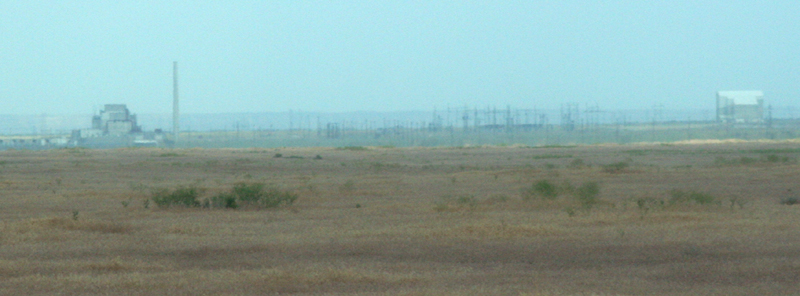 |
This is the B Reactor, and the cocooned C reactor, as
they appear from the road outside of the site. |
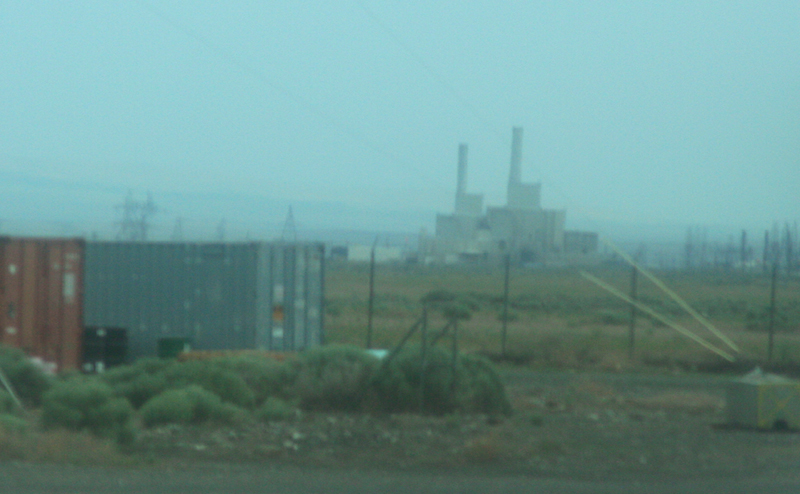 |
Left:
These are the twin D and DR reactors, built during
the war, as seen from the site of the B Reactor.
Below:
the raised silo are at the end of the building identifies this as the
REDOX building. This was one of several chemical separation canyons. |
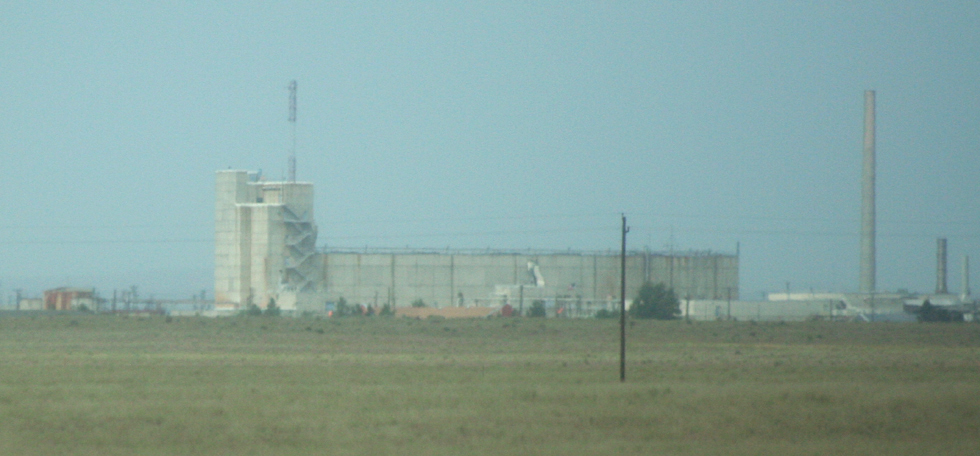 |
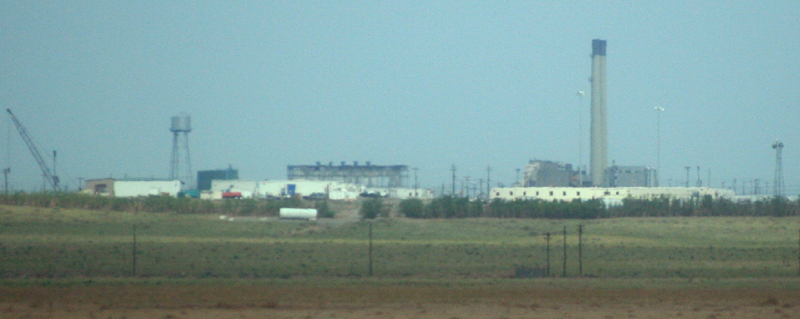 |
|
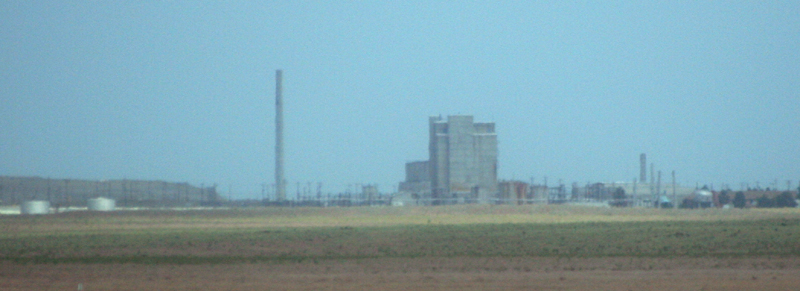 |
A look at the end of the REDOX building. |
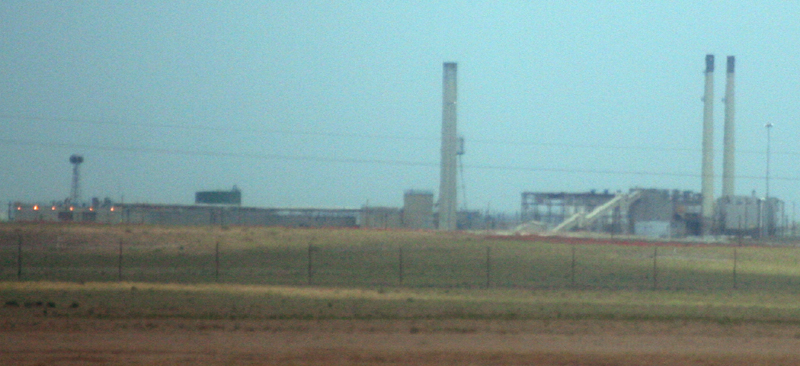 |
A T plant, as seen from the nearby highway. |
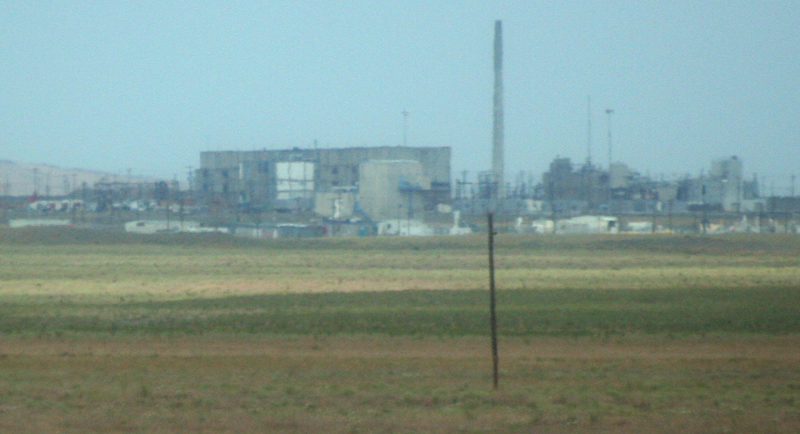 |
|
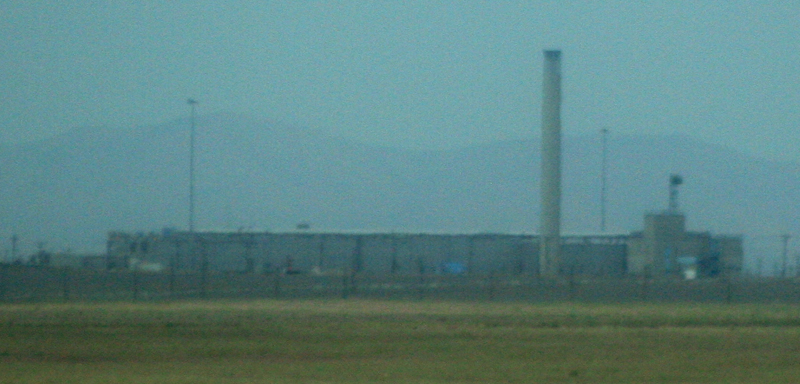 |
This appears to be the PFP complex, as seen from the
nearby highway. |
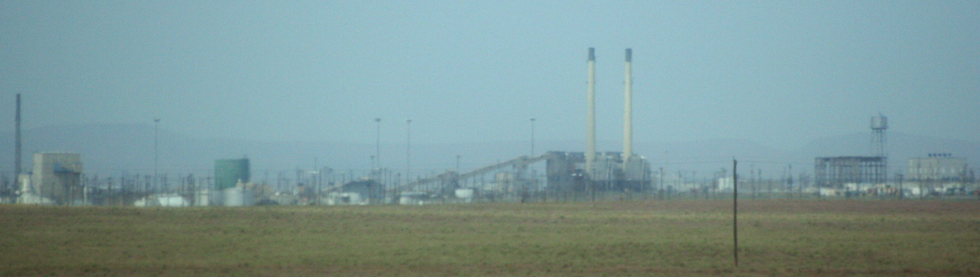 |
|