The Human Eye
Tube |
Lens |
Power |
Minimum Sensitivity |
Resolution |
Construction |
Type |
Cones and Rods |
13mm F 2.2 |
glucose |
0.046 lux to 0.1 lux |
130 megapixels |
biological |
all purpose |
The Versatile Eye
The first type of night vision
is simply the completely dark adapted human eye. Though this may sound
funny at first, the completely adapted eye, after thirty minutes or so,
sees much better in the dark than most people think. It continues to increase
in sensitivity, marginally, for as long as two hours in deep darkness.
This is the most underrated night vision device of them all. The human
eye is incredibly diverse, in part because of it's dual exposure system.
The eye contains cones and rods which are in effect, two separate systems,
though there is some overlap. Because of this, light perception of the
eye can increase by a factor of 10 billion from full sunlight to the least
light perceptible. The approximate dividing lines are daylight levels,
at which time the rods become saturated, and can no longer function, and
full moonlight, which is the dimmest light detectable by the cones. This
overlap, in which both rods and cones are used, is called mesopic vision.
Our daylight vision, in which we see detailed color images with great acuity,
is called photopic vision; it relies on the cones of the eye exclusively.
At low light levels, the cones are useless and we rely on the color blind
rods; this is called scotopic vision. These two systems coexist in the
human eye, being switched on and off as light levels require. Its almost
like having two sets of eyes, one for daylight, and one for night
A look at some of the other
specs of the eye leaves one pretty impressed. 130 megapixels is incredible
resolution, and .046 lux is better sensitivity than nearly all films and
video gear. This is said to be enough to detect a candle flame at 17 miles.
On top of all of this, the eye is self repairing, self focusing, and so
compact that two of them fit neatly into the front of our heads. They even
look nice.
The two systems
The eye uses small receptor cells to actually detect
light. These come in two types, are shaped somewhat
differently,
but share a similar internal structure. These are the rods, and the cones.
In both cases, these are long skinny cells, with two distinct sections.
The first section is the basic cell itself, complete with nucleus, and
all associated structures. The second section is called the outer segment,
and contains the cells specialized light gathering structures. The light
gathering outer segment contains a series of free floating discs containing
roughly 100 million molecules of light sensing chemicals. These discs,
as well as the cell membrane of the outer segment itself, fold back and
forth to increase their area. The chemicals imbedded in the discs also
fold back and forth, seven times, across the disk membrane itself. All
in all, the system is designed to expose as large a surface area as possible
to any light which might strike it.
The basic mechanism of the eye is chemical,
using a set of four related chemicals called opsins. These chemicals, as
a class are known as chromo-proteins. They consist of a light sensitive
pigment called a chromopore, combined with a protein called an opsin. all
are very similar in structure, and all tend to be long snakelike molecules.
There are four of them present in the human eye. Three of these are used
in concert, to provide color vision when light levels are high enough.
These three are sensitive to the following frequencies:
-
Blue (443 nm),
-
Green (535 nm)
-
Red (570 nm)
Each of these three chemicals is held in a special receptor cell called
a cone, so named because of it's shape. Since each cone holds large amounts
of only one of these chemicals, each is sensitive to only one color. The
nerve net, and ganglion in the retina produce the entire rainbow of colors
by mixing and interpreting the information from the three different types
of cones. The problem with this system is that it is not very sensitive
to light, requiring daylight levels to function properly. The cones offer
absolutely no night vision capacity at all. This is the photopic (daylight)
visual system.
There is a second system, the scotopic system,
which uses a different type of receptor cell, called a rod. Rods contain
a chromo-protein very similar to those contained in the cones. Rhodopsin
(also known as visual purple) is the photoreceptor in rod cells.
It absorbs best in the yellow-green (498 nm), which is why most night scopes
display in this color. Cone proteins are very similar to rhodopsin, in
fact, differing in only one key amino acid. This single difference in the
amino acid at position 122 results in the cone proteins resetting 100 times
faster than rhodopsin after absorbing light. This
makes the rods considerably slower and less acute than the cones, though
they are able to work with much lower levels of light. When discussing
night vision of the human eye we are dealing entirely with rhodopsin and
the rod receptors which contain it.
The average eye contains something like 120 million
rods, and 8 million cones. The cones tend to be
concentrated
in the center (fovea) of the retina. So concentrated are the cones in the
fovea, that it actually becomes a sort of a blind spot in scotopic (night)
vision. This is the reason for the old stargazers trick of looking off
to the side a bit, when viewing dim objects at night. During the day, while
the cones are usable, the fovea offers the best vision, the greatest resolution,
and finest acuity, due to the large numbers of cones concentrated there.
It is only in dim light, below the cones threshold of detection, that the
fovea goes blind. This is not to be confused with the classic blind spot
of the eye, which is the location of the nerve bundle which connects to
the optic nerve, and contains no light detecting cells at all.
The two systems compliment each other nicely.
Many animals with excellent daytime vision (birds in particular) can not
see at all during the hours of darkness. At the same time, most creatures
of the night hole up during the day as their eyes can not easily accustom
themselves to the light. Many hunters, cats in particular, share our dual
system, but it is by no means common. Incidentally, most animals also do
not share our range of focus. Humans have a focus range of something like
fifteen diopters. Most animals are limited to a few, or even a single diopter.
This tends to make most animals what we would consider to be nearsighted
or farsighted. The animals with the widest range are hawks and vultures
which can have as many as 50 diopters. As we age, we tend to lose this
range, becoming nearsighted or farsighted ourselves.
Night Vision (The Scotopic
system)
There are two major components
of dark adaptation namely dilatation of the pupil and the photochemical
alterations of the retina. The pupil can alter in size in response to light
by constricting and to the dark by dilating. The upper and lower limits
of pupil size are 3mm and 7mm. This variation is equal to over 500% alteration
in light entering the pupil from fully constricted to fully dilated. Although
not adequate to achieve full dark and light adaptation, pupil size alters
rapidly and gives, within 15 to 20 seconds, an appreciable increase in
the ability to see in dim light.
The secret here, is the
chemical rhodopsin, also known as visual purple, which is bleached out
of the eyes in the light, but begins to accumulate in the dark. This chemical
makes the rod sensors thousands of times more sensitive than the cones.
Rhodopsin is made up of opsins (the protein portion) and 11-cis-retinal
(a photosensitive chemical derived from vitamin A). One of the first processes
to take place is isomerization. This is caused by the reaction of light
photons with the 11-cis-retinal. Isomerization is a chemical process where
a compound is transformed into a different structure with the same chemical
configuration. When a rhodopsin molecule absorbs a photon it changes shape.
Each rhodopsin molecule
crosses the cell membrane of the portion of the rod known as the disk seven
times, winding it's way in and out. The light actually interacts with the
retinal. A photon hits a rod cell. In this cell there are 100 million molecules
of rhodopsin embedded in the membrane, the part of the cell furthest from
the cornea, in this portion of the cell the membrane forms a stack of disks.
In the dark, the retinal fits snugly into a binding pocket in the rhodopsin
molecule. When the retinal absorbs light it straightens out. This alters
the three-dimensional structure of the rhodopsin molecule activating it
and starting a biochemical cascade.
System vs Imager
Amazingly, a rod can be set
off by as little as one photon. This is incredible sensitivity, particularly
when considering that a photon is among the lightest of the subatomic particles.
Still, as with electronic NVD systems, the sensitivity of the imaging unit
(in this case the retina) is far greater than that of the system as a whole.
In the case of the retina, there are several factors reducing the usable
sensitivity. The main ones are:
-
Time
-
Scatter
-
Divergence
-
Noise
You might want to try a trick
some night. Dim the room lights, or go outside in the dark and bring a
small L.E.D. or other source of very dim light. if you move your head quickly,
you might notice that the light source seems to move at a different speed
than the surrounding dark objects. This is because the point light source
provides enough light to be seen by the cones, but the rest of the scene
is still being registered by the rods. Rods are much slower reacting than
cones. Though the reaction time of a rod is about 1/1000 of a second, it
takes something like 1/5 of a second for it to be restored to it's initial
state. Cones are able to do this up to 100 times faster. What this means
is that during the 1/5 of a second during which the rod is recovering,
any photon which strikes it will have no effect.
Less than half of the light
that enters the eye makes it to the retina. Even this is diminished, when
passing through the lens, by another half. So in a perfectly healthy eye
with no cataracts, pigmentation or other optical imperfections, only about
a quarter of the light striking the surface actually reaches the retina.
Divergence is the angle at which a photon strikes
the surface of the molecule. In order to affect the rod, light must hit
it at something less that a twenty degree angle. A more obtuse angle will
fail to excite the molecule.
Even after making it all
the way through the eye, and exciting a molecule of rhodopsin, there is
still one more obstacle to being perceived as a point of light; that is
the retina itself. The retina is actually equipped with a certain intelligence,
and ability to discriminate. It is saturated with an intricate nerve net,
and has it's own ganglia, giving it a certain amount of computing power.
Unlike any other sensory organ, the eyes are actually considered to be
part of the Central Nervous System, because to a certain extent, they can
think. One of the things that the ganglia in the retina look for are false
signals. At any given time, perhaps one in a hundred molecules of rhodopsin
change spontaneously. In order to filter out these false alarms, the nerve
net in the retina ignores solitary signals, and only "sees" signals when
adjacent rods are firing. At least, this is what is thought. In truth,
there is considerable doubt as to how this discrimination is done, and
how colors are mixed and perceived, but it is thought that this all takes
place in the retina, rather than in the brain.
Ultimately about ten percent
of the photons entering the eye actually make it to the rods, and strike
a molecule of rhodopsin in such a way as to cause a reaction. This is about
the same efficiency as modern NVD systems.
The details of the mechanism
In greater detail: Rod cells
are long hot dog affairs about 1/125 inch long with the synaptic and nucleus
end towards the incoming light. Each rod cell contains 2000 stacked
disks which contain up to 100 million modules of light sensitive pigment
rhodopsin. Each rhodopsin molecule has two parts, the opsin protein
and the light absorbing substance retinal, derived from vitamin A.
Before light hits it, the retinal is in the isomer form 11-cis-retinal.
When a packet of light energy is absorbed by rhodopsin, it twists 11-cis-retinal
to form another isomer, all-trans-retinal. This changes the configuration
of the opsin protein converting the whole molecule from rhodopsin into
metarhodopsin II in 1/1000 sec. Each metarhodopsin II molecule activates
hundreds of molecules of transducin, a protein. Each of these activates
an enzyme, phosphodiesterase, which alters the structure of thousands of
molecules of the neurotransmitter cGMP, cyclic guanosine monophosphate.
Levels of cGMP which in darkness are high, are thus reduced, closing channels
which allow sodium ions to flow through the cell membrane. cGMP's
function is to keep these channels open. In darkness, these open
channels allow the flow of positively charged sodium ions, called the dark
current, to counter the diffusion of positive potassium ions out of the
cell, making the inside slightly negative. When light hits the rod
cell, sodium entry is reduced, charging the cell's interior more negative,
called hyperpolarization. Hyperpolarization reduces the release of
neurotransmitters from synaptic vesicles, resulting in signals being sent
to the brain. All chemicals are cycled within 1/5 second.
It can be seen, then, that
the rods and cones are constantly signaling, and that it is only when they
are made to stop their signals that a point of light is registered. This
backward signaling is what researchers call dark light. This explains why
a knock on the head, rubbing of the eyes, certain drugs, or types of trauma
make us see spots, stars, and "fish". When signals are blocked, receptors
are damaged, or shocked, and no longer work, we interpret these lost signals
or functions as light.
Why it takes time to adapt to
the dark
In the case of the photopic
system, functionality depends upon having sufficient light levels to allow
the cones to operate. When there is too little light, the cones can no
longer respond to it. This seems obvious, and makes perfect sense, but
what shuts down the scotopic system, when light levels are too high, and
keeps our rods from dazzling us? It turns out that there is a chemical
switch.
The mechanism of dark adaptation
involves an interplay between Rhodopsin, Retinene and Vitamin A, all of
which are located in the rods of the retina. Rhodopsin (also known as visual
purple) is a photosensitive protein which is able to accumulate in the
rods during exposure to darkness. Rhodopsin is converted to Retinene on
exposure to the dimmest of light. During this interaction with light chemical
changes are made, which the brain "sees" as light. Retinene is an unstable
molecule and will spontaneously convert back to Rhodopsin if the light
exposure was of short duration (< 5 minutes) and there is sufficient
Oxygen and glucose available. This conversion back to Rhodopsin is rapid
and does not appreciably reduce dark adaptation. If however the exposure
to light is of long duration (>7 minutes), even dim light, then the Retinene
is converted to Vitamin A. Vitamin A is a stable molecule and the regeneration
of Rhodopsin from Vitamin A is slow and is accomplished over a number of
minutes. Thus prolonged exposure to light (>7 minutes) is required to significantly
alter dark adaptation (Campbell et al. 1955).
During the day, or during
constant exposure to moderate light levels and above, Rhodopsin is constantly
being bleached out, by the light, into it's vitamin A form, and never really
has a chance to accumulate. Thus the rods are essentially useless during
the day. A period of time lasting from thirty minutes to two hours is required
to allow rhodopsin levels to build to usable quantities. Once this happens,
the eyes are night adapted. This switching on and off is a protection and
prevents the visual system from being dazzled. It can take a few
minutes for the rhodopsin to completely bleach out, which is why our eyes
sometimes hurt when the light is switched on, or we enter a brightly lit
room after being in the darkness. In this case, huge amounts of Rhodopsin
are being converted at once, causing large numbers of rod receptors to
signal all at the same time. Our visual system is overloaded, and our eyes
hurt, and strain. After a few moments, the rhodopsin is bleached out, and
continues to be converted to vitamin A as soon as it is formed, in small
doses, rather than one big dose. The eyes can deal with this quite easily,
and are no longer overloaded.
Interestingly, red light
does not bleach out rhodopsin. This is why it is so useful for preserving
night vision. During the Second World War, submarine lookouts used to spend
the last hour or two before going on night watch, wearing red goggles,
to allow their eyes to get used to the dark. Often, red lights will be
used as night lighting for the same reason.
Alcohol and smoking
As they do with most other
things, alcohol and smoking impair night vision. They do so to a surprising
degree. Smoking in particular is the great destroyer of night vision. Non
smokers in a study, were able to detect a light source of 46 microlux,
while dark adapted smokers could not detect light below 69 microlux, an
almost 50% increase above non smoker thresholds. Note that this comparison
was made during abstinence from smoking materials. Under conditions of
carbon monoxide exposure (as while smoking) there was no significant increase
in
threshold
of non smokers, however in smokers the average threshold increased even
more, to 100 microlux. This is over double that of the non smokers. A good
video camera has a sensitivity of 0.1 lux (100 microlux), the human eye
in a smoker is thus only as sensitive as a good video camera. This is primarily
attributed to smoking's effect of decreasing blood flow, and oxygen, while
raising carbon monoxide levels in the body. This makes it more difficult
and time consuming for the resynthesis of visual purple in the rod receptors.
As was mentioned in the preceding section, visual purple reconstitutes
itself naturally in the presence of sufficient glucose, and oxygen.
Alcohol reduces the ability
to see faint objects by decreasing the light entering the eye by up to
20% and then reducing the ability of the brain to fully appreciate contrast.
It is also possible that the ganglia, along with the rest of the extensive
nerve net in the retina can become "drunk", as well as the brain. The pupil
never fully dilates in a chronic drinker, or in a social drinker who happens
to be drinking. Drink also significantly increases the time it takes for
the eyes to become completely night adapted.
Night vision also decreases
somewhat with age. In part this is because of the tendency of the lens
to begin to yellow as the eye grows older. This decreases somewhat the
amount of light allowed to enter the eye. As with most of the rest of the
body, the efficiency of the eye, and the volume of blood reaching it decreases
as the body ages. This affects the ability to produce rhodopsin (visual
purple) quickly. Even so, a young man who smokes will have poorer night
vision than a middle aged to old man who does not. The human body is designed
with considerable margins. It has numerous redundant systems, and most
are rarely ever pushed to anything like their design limits. The night
adapted human eye is different, and works very close to it's limits. The
lungs, heart, and even the brain may be able to compensate out of their
excess capability, as can the eyes during regular photopic (daylight) vision.
The night adapted eyes, working so close to the limit, can not; there is
no excess to work with, so that any detrimental factor immediately begins
to impair function. So don't smoke, it'll stunt your night vision.
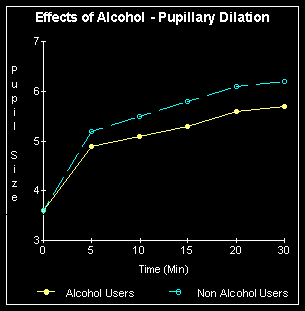